As the media, politicians and climate activists continue to circulate hysterical hot air from the Cop26 conference, the topic of climate change or anthropogenic global warming (AGW) has become an emotional one, increasingly detached from the thoughtful and meticulous process of theory development, calculation and observation that is supposed to characterise scientific endeavour.
It may come as a surprise to some that “The Science”, as expounded in the IPCC Summaries for Policymakers that inform conference participants, is not uncritically accepted by all scientists in the field, and that widely different views are held by a substantial cadre of experienced and eminent researchers. Moreover, a multitude of peer-reviewed papers contradict many aspects of the IPCC’s alarmist narrative. Furthermore, a coherent theory about the impact of changes in greenhouse gases (GHGs) is starting to emerge, one that is built up from the underlying physics, rather than extracted from fanciful computer simulations. My aim here is to highlight some of the relevant papers and to inform any motivated layman who wishes to explore outside the dogmatic strictures of the mainstream narrative.
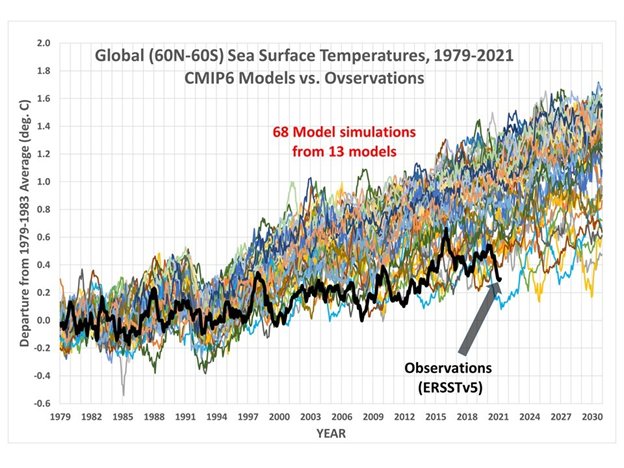
Let us start with an irrefutable example of the inability of climate models (general circulation models, GCMs) to provide meaningful projections. Climatologist and former NASA scientist Dr Roy Spencer compares observed global sea surface temperatures with those predicted by climate models and provides an analysis (Fig.1). This shows unequivocally that warming is occurring much more slowly than the average model says it should. Indeed, it can be seen by simple inspection that the climate models currently in use by the IPCC (CMIP6) exaggerate observed warming by a factor of up to around five times.
A key determinant of the warming in the CMIP models is the assumption made about the sensitivity of surface temperatures to atmospheric CO2 concentrations. This ratio is often described by an Equilibrium Climate Sensitivity (ECS), which varies between a 2.6°C and 8.5°C increase per doubling of CO2, depending on the IPCC scenario.
Similar discrepancies to those in Fig.1 arise if one compares the outputs of models with satellite records of atmospheric temperatures. The climate models also fail to replicate the temperature profile of the atmosphere vertically, predicting a hot spot in the tropical upper troposphere that is simply not observed [McK, Mit.]. The very least that any reasonable person should conclude is that the climate models are unsuitable for policymaking and that the IPCC’s proposals are not well-founded in science.
The factors contributing to the climate models’ lack of realism are discussed in Dr Steven Koonin’s recent book, Unsettled. The IPCC models contain very simplified approximations to the underlying physics and depend on tuning (via ad hoc parameters) to obtain results that are deemed acceptable to the IPCC. Moreover, there is the fundamental concern that the underlying physics built into the models (via their structure and parameters) may be incomplete or wrong. To explore this line of reasoning, we need to delve into atmospheric physics.
The atmosphere is described by an equilibrium between convection (the upward movement of warm air) and radiation (the emission of heat and energy) [Man.]. In the troposphere (typically up to around 11 kilometres altitude), convection dominates, because hot air rises, and this process transfers heat from the Earth’s surface more effectively than the infrared radiation which it emits. The physics of convection follow from the application of well-understood laws of gas dynamics to an atmosphere in equilibrium and subject to the Earth’s gravity. They determine how temperatures (as well as air pressure and density) decrease as you go up higher. The key parameters that determine the rates at which temperatures change with altitude (termed ‘lapse rates’) are the technical properties of the atmosphere, such as its specific heat, the latent heat of its water vapour content (which varies depending on temperature), and the strength of gravity. Local temperature gradients (how temperature changes with height) are limited in magnitude by the lapse rate of dry air (9.8°C per kilometre of altitude), and are often approximated by meteorologists by a lower ‘environmental lapse rate’ of 6.5°C/km that reflects the typical humidity of the air. Under this environmental lapse rate, temperatures fall from an average of around 15°C at the surface to minus 56.5°C at the tropopause altitude of 11 km (where the troposphere ends). Importantly, the tropospheric lapse rate is independent of the concentrations of greenhouse gases like CO2 (other than water vapour) due to their low concentrations.
In the stratosphere (which extends from the tropopause up to the stratopause at 47 km), the atmosphere is less dense, convection ceases, and radiation effects (involving both infrared-active greenhouse gases such as CO2 and interactions between ozone and ultraviolet light) become important factors in the temperature gradient. These cause stratospheric temperatures to increase from the tropopause up to close to 0°C at the stratopause.
Above the stratopause lies the mesosphere. This extends up to the mesopause at 86 km, which marks a practical top-of-atmosphere for the purposes of atmospheric thermodynamics.
In order for overall atmospheric temperatures to be in equilibrium, the Law of Conservation of Energy requires that the amount of heat energy radiated to space from the Earth’s climate system (comprising land and ocean surfaces, clouds, greenhouse gases), taken together with the energy (mainly light) reflected by the surface and clouds, should balance the energy reaching the Earth from the Sun. After accounting for the radiation reaching the Earth from the Sun, minus the energy reflected, this requires that the infrared radiation from the Earth to space should be radiated from surfaces and infrared-active gases that have an average temperature of about minus 18°C.
The average temperature difference of about 33°C between the land and ocean surfaces at 15°C and the infrared radiation out to space at minus 18°C is termed the greenhouse effect. The greenhouse effect depends on the manner in which radiation from the surface is modified by greenhouse gases and by clouds in the atmosphere.
In the absence of clouds, some infrared radiation from the surface is absorbed by greenhouse gases in the lower atmosphere, before being emitted to space by greenhouse gases at a higher altitude. The difference in temperature due to altitude means that less energy is radiated to space than by the surface. The balance (termed ‘radiative forcing’) remains part of the climate system, where it contributes to convection, cloud formation, winds, and other atmospheric dynamics. Importantly, the rate of absorption of radiation depends on the frequency of the radiation, with some parts of the infrared spectrum being unaffected, while others are heavily absorbed. Quantifying the extent to which changes in greenhouse gas concentrations affect radiative forcing (and thus the atmospheric temperature profile) is far from trivial, but increasingly precise calculations have been carried out by atmospheric physicists.
It should be mentioned that energy fluxes, which are impacted by radiative forcing, can be related to temperatures by the black body radiation law, which states that the energy flux (measured in watts per square metre) from an idealised surface is equal to the fourth power of its temperature (measured in degrees Kelvin) times the Stefan Boltzmann constant. Typical discussions of the greenhouse effect involve both fluxes and temperatures. The technical conversions are omitted in this note in the interests of brevity.
Early attempts to quantify the effects of varying CO2 and other greenhouse gases include Manabe and Wetherald [Man.], who calculated in 1967 that a doubling of CO2 levels would lead to a surface temperature increase of around 2°C. Specifically, they found an all-sky ECS for CO2 of between 1.3°C and 2.4°C (depending on whether the humidity profile of the atmosphere is fixed in terms of absolute or relative humidity). Such parameters were incorporated into the early climate models being developed at that time. It was, however, infeasible to make systematic measurements to validate calculated atmospheric temperatures and radiation fluxes prior to the extensive deployment of satellites some decades later.
More recently, in 2020, physicists Wijngaarden and Happer conducted precise radiative calculations for cloud-free skies, considering the quantum absorption lines of the main greenhouse gas molecules recorded in the HITRAN database (totalling around a third of a million lines) and the balance of absorption and emission for altitudes from sea level up to an effective top-of-atmosphere at the mesopause altitude of 86 km [W&H]. Their calculated intensities of the spectrum of infrared radiation out to space from the climate system are in strong agreement with measurements of radiation intensities taken from satellites. Their findings confirm a clear-sky ECS for CO2 in the range of 1.4°C to 2.3°C, of comparable magnitude to that of Manabe and Wetherald [Man.]. They also show that the forcing from a doubling of methane or nitrous oxide is a factor of three to four times smaller (due to the overlap of their absorption lines with water vapour and CO2) and, furthermore, that radiative forcing in clear skies depends strongly on latitude (being positive in tropical and temperate regions, but negative at the wintertime pole). Lastly, they demonstrate that radiative forcing is negative in the stratosphere (negative forcing is where the upper atmosphere is warmer than the layer below and so emits more infrared radiation, balanced by other heat flows). Summaries of Wijngaarden and Happer’s findings are available in a form accessible to non-experts, for example, in video presentations by Professor Will Happer [Hap] and by Dr Tom Sheahen [She].
From Wijngaarden and Happer’s work it is clear that there is no basis in the underlying radiation physics for using the higher ECS values for CO2, varying from 2.6°C up to 8.5C, that are deployed in the IPCC models and presented to the media and public. It’s also clear that methane is not the threat presented by the IPCC, and that we should not expect the wintertime poles to warm due to human greenhouse gas emissions.
Wijngaarden and Happer emphasise that these are clear sky calculations. At any time, two thirds of the sky is covered by clouds, so it makes sense also to ask about the atmospheric physics of cloudy skies.
Clouds are largely opaque to the infrared radiation from below and radiate across the infrared spectrum according to their temperature. In principle, since cloud temperatures are determined by their altitude and the lapse rate, we can, for the purpose of radiative forcing calculations, treat the clouds as an extension of the surface of the Earth, and simply consider the radiative forcing that arises between the cloud top surfaces and the relevant greenhouse gases higher up in the atmosphere. Of course, the temperature difference between the cloud-tops and the upper atmosphere is smaller, and greenhouse gas densities are far lower, so one can expect radiative forcing above cloud surfaces to be considerably reduced from that above ground level in clear skies.
Unfortunately, precise calculations are hampered by the complications of many different types of cloud, which may be formed from water droplets or ice crystals, and have varying altitudes, depths and opacities.
The effects of cloud cover are, however, studied empirically in a recent paper by Dübal and Vahrenholt [D&V]. Working with energy flux data generated by NASA’s CERES satellite system, they show that, over the period 2000 to 2020, the driver of surface warming has been a reduction in cloudiness, while there has been no increase in radiative forcing in cloudy skies. These findings are in conflict with the IPCC narrative that surface warming is primarily due to radiative forcing.
Moreover, Dübal and Vahrenholt identify that the radiative forcing above cloudy skies (from cloud-tops with an average altitude of 5 km and an average temperature of minus 16°C, up to the CERES satellite measurement altitude of 20 km) is only 33 watts per square metre, as compared with 130 watts per square metre for clear skies. Based on the foregoing, the relative contributions (implicit in the CERES data) of the various greenhouse gases and clouds to the overall all-sky greenhouse effect of 35°C can be separated out as in Table 1 below.
Clear Skies | Cloudy Skies | All-Sky | |
Proportion | 33% | 67% | 100% |
Greenhouse Effect Due To: | |||
Clouds | – | 32°C | 22°C |
Radiative Forcing | 27°C | 6°C | 13°C |
Total | 27°C | 38°C | 35°C |
Of which: Clouds & Water Vapour | 61% | 94% | 85% |
It can be seen that the great majority, 22°C of the 35°C all-sky greenhouse effect is attributable to the temperature lapse from the surface up to the cloud tops. Radiative forcing averaging 13°C mainly arises in clear skies and is dominated by water vapour. The net result is that water vapour and clouds taken together account for 85% of the greenhouse effect with the balance accounted for by CO2 (10%) and the minor greenhouse gases (5%).
In terms of the underlying physics, the greenhouse effect at ground level decomposes into two mechanisms, comprising (1) radiative forcing above surfaces (ground/ocean in clear skies or cloud tops) and (2) the temperature difference between the surface and clouds, which is determined by cloud altitudes and the lapse rate. As noted earlier, the lapse rate results from the thermodynamics of gases in Earth’s gravity, and is independent of the concentrations of CO2 and the minor greenhouse gases. Such surface warming relative to a cloudy atmosphere arises on any planet, irrespective of greenhouse gases, as was explained by physicist Hans Jelbring in 2003 [Jelb.].
It is worth commenting that the CERES satellite system uses an altitude of only 20 km to measure its top-of atmosphere fluxes, compared with the 86 km top-of atmosphere studied by Wijngaarden and Happer, so CERES misses some of the negative radiative forcing in the stratosphere, and its radiative forcings may be exaggerated. If we increase the measurement altitude to consider the situation at the mesopause (86 km), the forcings identified by Dübal and Vahrenholt can be expected to reduce by around 12 watts per square metre (as per Figure 7 in [W&H]). Thus, we can estimate that the radiative forcing between cloud-tops and the top-of-atmosphere is only around 10-20% of that in clear skies. Given that two-thirds of skies are cloudy, this in turn implies an average all-sky radiative forcing of only 40-45% of the clear-sky forcing and would suggest an all-sky ECS for CO2 in the range of 0.6°C to 1.0°C.
Such estimates are well below the bottom of the ECS range in the IPCC’s CMIP6 model set and we must ask whether these can be corroborated.
So, let’s turn to a novel paper by Coe et al. [Coe], who approach the question of the all-sky greenhouse effect through a simplified analysis of the overall climate system. They start from a calculation of clear-sky absorption of surface radiation based on the HITRAN database and then find the overall factor (for re-emission to space of absorbed radiation) which yields the observed all-sky greenhouse effect. This generates a simple equation between average surface temperatures, clear-sky absorption by greenhouse gases, and the overall re-emission factor (which implicitly includes the effect of clouds). A perturbation analysis in response to changes in greenhouse gas concentrations generates an estimated ECS for CO2 of only 0.5°C. Coe et al. also find that the effects of doubling methane and nitrous oxide are negligible due to the prior saturation of absorption lines by the greenhouse gas content of the atmosphere. Their analysis attributes 90% of the greenhouse effect to water alone, whether in the form or clouds or water vapour. It would imply that the amount of anthropogenic global warming (since the Industrial Revolution) due to CO2 was only 0.25°C.
It should be noted that the Coe et al. calculations yield only first order sensitivities of the climate system to changes in greenhouse gases, due to their assumption of a fixed re-emission factor. However, they establish an interesting benchmark until a more refined analysis of the all-sky problem, including clouds, becomes available. The sensitivities to atmospheric CO2 under these various approaches are compared in Table 2.
ECS for CO2 | Sky type | |
IPCC/CMIP6 | 2.6 to 8.5 | all sky* |
Manabe & Wetherald (1967) | 1.4 to 2.9 | clear sky |
Manabe & Wetherald (1967) | 1.3 to 2.4 | all sky |
Wijngaarden & Happer (2020) | 1.4 to 2.3 | clear sky |
All-sky model as above | 0.6 to 1.0 | all sky |
Coe et al. (2021) | 0.5 | all sky |
As a corollary, there is a large natural component in the variability of surface temperatures that is unrelated to human emissions. Over longer time scales this is obvious, given the climate record, which includes ice ages, the Mediaeval Warm Period and the Little Ice Age. Over shorter time horizons, there are numerous natural cycles being investigated to explain climate variability, such as the mid-20th century peak in temperatures. These cycles include the Atlantic Multidecadal [D&V] and Pacific Decadal Oscillations, as well as cycles in solar activity [Lea.] [Con.] and variations in cloud structure.
The question of how much the Sun has influenced Northern Hemisphere temperature trends was examined in depth in a recent paper co-authored by a multi-disciplinary group of 23 scientists [Con.]. This paper contests the IPCC conclusion that variations in solar activity are largely irrelevant to climate, and enumerates the many studies that find a relationship between solar activity (in terms of luminosity, magnetic activity and/or sunspots) and climate. The mechanisms under study include variations in total solar irradiance (which affects temperatures directly), variations in ultraviolet irradiance (which affect the upper atmosphere), and variations in magnetic fluxes (which affect inbound cosmic rays and the process of cloud formation) [Sven.]. Connolly et al. also examine the urban heat island effect and the manner in which it biases the temperature time series used by the IPCC. An important conclusion is that variations in solar activity may, given the uncertainties in our present understanding of climate dynamics, plausibly account for a majority of the warming observed since the Little Ice Age. Connolly et al. argue that it is premature, before resolving such matters, for the IPCC to promote conclusions about man-made global warming to policymakers. A recent presentation by Dr Willie Soon [Soon] expounds some of these issues.
In short, no scientist who studies the range of scientific literature can reasonably claim that the subject of influences on the climate is remotely ‘settled’. The reality is that a multiplicity of factors are at work, and so, by focusing on human emissions, it appears that the IPCC has, through ‘force fitting’ between its selectively chosen historic global temperature estimates and the inadequately structured and parameterised CMIP models, reached a highly exaggerated view of climate sensitivity to CO2. Specifically, the range of ECS values for CO2 adopted by the IPCC overstates those obtained from a physics analysis of causal mechanisms, consistent with satellite measurements, by a factor of up to five to 17 times.
In another recent paper, climate scientist Kerry Emanuel [Em.] argues that “simulation without understanding imperils scientific progress and, paradoxically, may impede the development of better models”. Based on the review herein, it is suggested that an improved understanding of physics, and a study of the relevant papers that are systematically ignored by many climate modellers, would help the climate community fix its broken models.
Several of the shortcomings of IPCC reports lead back to clouds, which many climate scientists admit are poorly understood. Key issues include the difference between clear-sky and cloudy-sky radiative forcing, and the response of clouds to solar activity, neither of which appear to have been satisfactorily incorporated into climate models. Are there cloudy skies ahead for dogmatic climate alarmism?
Dr. Rudolph Kalveks is a retired executive. His PhD was in theoretical physics.
Bibliography
- [Coe] David Coe, Walter Fabinski, Gerhard Wiegleb. “The Impact of CO2, H2O and Other ‘Greenhouse Gases’ on Equilibrium Earth Temperatures.” International Journal of Atmospheric and Oceanic Sciences. Vol. 5, No. 2, 2021, pp. 29-40.
- [Con.] Connolly, R., Soon, W., Connolly, M., Baliunas, S., Berglund, J., Butler, C.J., Cionco, R.G., Elias, A.G., Fedorov, V.M., Harde, H. and Henry, G.W., 2021. “How much has the Sun influenced Northern Hemisphere temperature trends? An ongoing debate.” Research in Astronomy and Astrophysics, 21(6), p.131.
- [D&V] Dübal, H.R. and Vahrenholt, F., 2021. “Radiative Energy Flux Variation from 2001–2020.” Atmosphere, 12(10), p.1297.
- [Em.] Emanuel, K., 2020. “The relevance of theory for contemporary research in atmospheres, oceans, and climate.” AGU Advances, 1(2), p.e2019AV000129.
- [Jelb.] Jelbring, H., 2003. “The ‘Greenhouse Effect’ as a Function of Atmospheric Mass.” Energy & Environment, 14(2-3), pp.351-356.
- [Lea.] Leamon, R.J., McIntosh, S.W. and Marsh, D.R., 2021. “Termination of solar cycles and correlated tropospheric variability.” Earth and Space Science, 8(4), p.e2020EA001223.
- [Man.] S. Manabe and R. T. Wetherald. “Thermal Equilibrium of the Atmosphere with a Given Distribution of Relative Humidity.” J. Atmos. Sci. 24, 241 (1967).
- [McK] McKitrick, R. and Christy, J., 2020. “Pervasive warming bias in CMIP6 tropospheric layers.” Earth and Space Science, 7(9), p.e2020EA001281.
- [Mit.] Mitchell, D. M., Eunice, Y. T., Seviour, W. J. M., Haimberger, L. & Polvani, L. M. 2020. “The vertical profile of recent tropical temperature trends: Persistent model biases in the context of internal variability.” Environ. Res. Lett., 15.
- [Sven.] Svensmark, H., Svensmark, J., Enghoff, M.B. and Shaviv, N.J., 2021. “Atmospheric ionization and cloud radiative forcing.” Scientific Reports, 11(1), pp.1-13.
- [W&H] van Wijngaarden, W.A. and Happer, W., 2020. “Dependence of Earth’s Thermal Radiation on Five Most Abundant Greenhouse Gases.” arXiv preprint arXiv:2006.03098.
- [Hap] ] Prof. William Happer, “How to Think About Climate Change.” CO2.
- [She] Dr Tom Sheahen, “Applying the Scientific Method Shows Why Climate Models Run Hot.” YouTube.
- [Soon] Dr Willie Soon, “Studying the Role of the Sun on Climate.” YouTube.